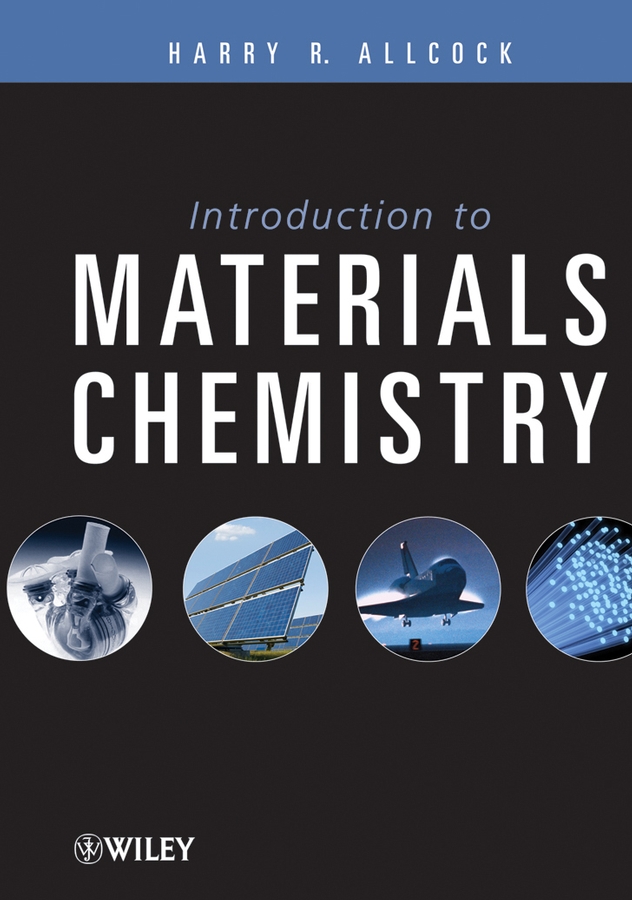
Contents
Preface
Part I Introduction to Materials Science
1 What is Materials Chemistry?
A. DIFFERENT TYPES OF MATERIALS
B. USES OF MATERIALS
C. APPROACHES TO PRODUCING NEW MATERIALS, NEW PROPERTIES, AND USES
D. DEVICES AND MACHINES
E. THE ROLE OF CHEMISTRY IN MATERIALS SCIENCE
F. A BROADER PERSPECTIVE
G. TERMINOLOGY
H. EXAMPLE JOURNALS WHERE MATERIALS SCIENCE PUBLICATIONS CAN BE FOUND
I. STUDY QUESTIONS (for class discussions or essays)
2 Fundamental Principles that Underlie Materials Chemistry
A. WHY ARE DIFFERENT MATERIALS DIFFERENT?
B. THE ROLE OF DIFFERENT ELEMENTS
C. DIFFERENT TYPES OF CHEMICAL BONDS
D. SIZE OF MOLECULAR UNITS
E. DIFFERENT SHAPES OF COMPONENT MOLECULES AND INFLUENCE ON SOLID-STATE STRUCTURE
F. SUGGESTIONS FOR FURTHER READING
G. STUDY QUESTIONS (for class discussions or essays)
3 Basic Synthesis and Reaction Chemistry
A. UNDERLYING PRINCIPLES
B. STARTING POINTS FOR MATERIALS SYNTHESIS—ISOLATION OF ELEMENTS
C. PRINCIPLES THAT UNDERLIE MATERIALS SYNTHESIS
D. ILLUSTRATIVE CHEMISTRY OF SELECTED NONMETALLIC ELEMENTS
E. SUGGESTIONS FOR FURTHER READING
F. STUDY QUESTIONS (for class discussions or essays)
4 Structure Determination and Special Techniques for Materials Characterization
A. PURPOSE
B. ANALYSIS OF BULK MATERIALS
C. SURFACE AND THIN-FILM ANALYSIS TECHNIQUES
D. SOLUTION ANALYSIS TECHNIQUES
E. SUGGESTIONS FOR FURTHER READING
F. STUDY QUESTIONS (for essays or class discussions)
Part II Different Types of Materials
5 Small Molecules in Solids
A. IMPORTANCE OF SMALL-MOLECULE MATERIALS
B. PACKING OF SMALL MOLECULES IN THE SOLID STATE
C. SELF-ASSEMBLY BY CRYSTALLIZATION
D. SPHERICAL MOLECULES SUCH AS FULLERENES IN THE SOLID STATE
E. DISK-SHAPED MOLECULES AND OTHER FLAT STRUCTURES
F. ROD-SHAPED MOLECULES
G. CHARGE TRANSFER COMPLEXES
H. CLATHRATES—MOLECULAR INCLUSION ADDUCTS
I. SUGGESTIONS FOR FURTHER READING
J. STUDY QUESTIONS (for class discussions or essays)
6 Polymers
A. OVERVIEW
B. SYNTHESIS OF POLYMERS
C. STRUCTURE–PROPERTY RELATIONSHIPS AND POLYMER DESIGN
D. POLYMERS IN THE SOLID STATE
E. FABRICATION OF POLYMERS
F. EXAMPLE POLYMERIC MATERIALS
G. FUTURE CHALLENGES IN POLYMERIC MATERIALS SCIENCE
H. SUGGESTIONS FOR FURTHER READING
I. STUDY QUESTIONS (for class discussions or essays)
7 Glasses and Ceramics
A. OVERVIEW
B. OXIDE CERAMICS AND GLASSES OBTAINED OR PRODUCED DIRECTLY FROM MINERALOGICAL MATERIALS
C. OXIDE CERAMICS FROM SMALL-MOLECULE INORGANIC AND ORGANOMETALLIC PRECURSORS
D. NONOXIDE CERAMICS
E. FABRICATION OF CERAMICS AND GLASSES
F. FUTURE CHALLENGES IN CERAMICS AND GLASS SCIENCE
G. SUGGESTIONS FOR FURTHER READING
H. STUDY QUESTIONS (for class discussions or essays)
8 Metals
A. IMPORTANT ASPECTS OF METAL SCIENCE AND TECHNOLOGY
B. ISOLATION OF SPECIFIC METALS FROM THEIR ORES
C. CORROSION
D. SOLID-STATE STRUCTURE OF METALS AND ALLOYS
E. ELECTRICAL CONDUCTIVITY
F. THE COLOR OF METALS
G. THERMAL CONDUCTIVITY OF METALS
H. MAGNETIC PROPERTIES OF METALS
I. MECHANICAL PROPERTIES OF METALS
J. FABRICATION OF METALS
K. FUTURE CHALLENGES IN METALLIC MATERIALS
L. SUGGESTIONS FOR FURTHER READING
M. STUDY QUESTIONS
9 Alloys, Composites, and Defects
A. OVERVIEW
B. PURE MATERIALS AND HOMOGENEOUS SOLID SOLUTIONS
C. HETEROPHASE MATERIALS
D. SUGGESTIONS FOR FURTHER READING
E. STUDY QUESTIONS
Part III Materials in Advanced Technology
10 Semiconductors and Related Materials
A. IMPORTANCE OF SEMICONDUCTORS
B. SEMICONDUCTOR THEORY
C. PREPARATION OF SEMICONDUCTOR-GRADE SILICON AND COMPOUND SEMICONDUCTORS
D. ORGANIC POLYMER SEMICONDUCTORS
E. PHOTOLITHOGRAPHY AND MICROLITHOGRAPHY
F. PHOTORESISTS
G. ELECTRON BEAM LITHOGRAPHY
H. X-RAY LITHOGRAPHY
I. CIRCUIT WIRING
J. SEMICONDUCTOR DEVICES
K. UNSOLVED PROBLEMS IN SEMICONDUCTOR MATERIALS SCIENCE
L. SUGGESTIONS FOR FURTHER READING
M. STUDY QUESTIONS (for class discussions or essays)
11 Superconductors
A. OVERVIEW
B. NOMENCLATURE
C. SYNTHESIS OF HIGH-TEMPERATURE SUPERCONDUCTORS
D. SOLID-STATE STRUCTURE
E. THEORIES OF SUPERCONDUCTION
F. OTHER SUPERCONDUCTING SYSTEMS
G. CURRENT AND PROPOSED USES FOR SUPERCONDUCTORS
H. CHALLENGES FOR THE FUTURE
I. SUGGESTIONS FOR FURTHER READING
J. STUDY QUESTIONS (for class discussions or essays)
12 Solid Ionic Conductors: Advanced Materials for Energy Generation and Energy Storage
A. GENERAL OBSERVATIONS
B. FUEL CELL MATERIALS
C. BATTERY ELECTROLYTE MATERIALS
D. CAPACITORS AND SUPERCAPACITORS
E. CHALLENGES FOR THE FUTURE
F. SUGGESTIONS FOR FURTHER READING
G. STUDY QUESTIONS
13 Membranes
A. BACKGROUND
B. POROUS MEMBRANES
C. MEMBRANES THAT FUNCTION BY A CHEMICAL REACTION
D. NONPOROUS MEMBRANES THAT DO NOT REACT WITH PARTICIPATING MOLECULES
E. SPECIFIC EXAMPLES OF MATERIALS USED IN SOLID POLYMERIC MEMBRANES
F. GEL MEMBRANES
G. TESTING OF MEMBRANES
H. SOUND TRANSDUCER MEMBRANES
I. CHALLENGES FOR THE FUTURE
J. SUGGESTIONS FOR FURTHER READING
K. STUDY QUESTIONS (for essays and class discussions)
14 Optical and Photonic Materials
A. OVERVIEW
B. PASSIVE OPTICAL MATERIALS
C. RESPONSIVE OPTICAL MATERIALS
D. CHALLENGES FOR THE FUTURE
E. FINAL COMMENTS
F. SUGGESTIONS FOR FURTHER READING
G. STUDY QUESTIONS
15 Surface Science of Materials
A. PERSPECTIVE
B. SUMMARY OF CHARACTERIZATION METHODS
C. SURFACES OF METALS
D. CERAMIC SURFACES
E. POLYMER SURFACES
F. SURFACES OF SEMICONDUCTORS
G. ASSEMBLY OF MOLECULES ON SURFACES
H. ADHESION AND SURFACE CHEMISTRY
I. RELATIONSHIP TO OTHER MATERIALS TOPICS
J. SUGGESTIONS FOR FURTHER READING
K. STUDY QUESTIONS
16 Biomedical Materials
A. SPECIAL REQUIREMENTS FOR BIOMEDICAL MATERIALS
B. TRADITIONAL BIOMEDICAL MATERIALS
C. MATERIALS FOR SPECIFIC MEDICAL APPLICATIONS
D. FABRICATION AND TESTING OF BIOMEDICAL MATERIALS
E. UNSOLVED PROBLEMS IN BIOMEDICAL MATERIALS SCIENCE
F. SUGGESTIONS FOR FURTHER READING
G. STUDY QUESTIONS
17 Materials in Nanoscience and Nanotechnology
A. BACKGROUND AND MOTIVATION
B. SYNTHESIS AND FABRICATION OF NANOSTRUCTURES
C. EXAMPLES OF NANOSTRUCTURES
D. MAJOR CHALLENGES IN NANOSCIENCE AND TECHNOLOGY
E. SUGGESTIONS FOR FURTHER READING
F. STUDY QUESTIONS
Glossary
COLOR PLATE
Index
Copyright © 2008 by John Wiley & Sons, Inc. All rights reserved
Published by John Wiley & Sons, Inc., Hoboken, New Jersey
Published simultaneously in Canada
No part of this publication may be reproduced, stored in a retrieval system, or transmitted in any form or by any means, electronic, mechanical, photocopying, recording, scanning, or otherwise, except as permitted under Section 107 or 108 of the 1976 United States Copyright Act, without either the prior written permission of the Publisher, or authorization through payment of the appropriate per-copy fee to the Copyright Clearance Center, Inc., 222 Rosewood Drive, Danvers, MA 01923, (978) 750-8400, fax (978) 750-4470, or on the web at www.copyright.com. Requests to the Publisher for permission should be addressed to the Permissions Department, John Wiley & Sons, Inc., 111 River Street, Hoboken, NJ 07030, (201) 748-6011, fax (201) 748-6008, or online at http://www.wiley.com/go/permission.
Limit of Liability/Disclaimer of Warranty: While the publisher and author have used their best efforts in preparing this book, they make no representations or warranties with respect to the accuracy or completeness of the contents of this book and specifically disclaim any implied warranties of merchantability or fitness for a particular purpose. No warranty may be created or extended by sales representatives or written sales materials. The advice and strategies contained herein may not be suitable for your situation. You should consult with a professional where appropriate. Neither the publisher nor author shall be liable for any loss of profit or any other commercial damages, including but not limited to special, incidental, consequential, or other damages.
For general information on our other products and services or for technical support, please contact our Customer Care Department within the United States at (800) 762-2974, outside the United States at (317) 572-3993 or fax (317) 572-4002.
Wiley also publishes its books in a variety of electronic formats. Some content that appears in print may not be available in electronic formats. For more information about Wiley products, visit our web site at ww.wiley.com.
Library of Congress Cataloging-in-Publication Data is available.
Allcock, Harry R.
Introduction to Materials Chemistry
ISBN: 978-0-470-29333-1
Preface
Modern undergraduates and graduate students are keenly interested in the various ways in which chemistry impacts everyday life. This interest stems partly from career imperatives, but also from a natural interest in the relationship between fundamental science and the technology that increasingly dominates our lives. Classical chemistry courses at the university level rightly emphasize fundamental science. But courses that focus on the utilization of that chemistry are still rare.
This book is an outgrowth of a course in materials chemistry that I have taught for several years in the Chemistry Department at The Pennsylvania State University. It is a course taken by advanced undergraduates and by students who are in the first year of the graduate program in chemistry, most of whom have had little or no prior exposure to materials science. The class has also attracted undergraduates from materials science and chemical engineering programs who are seeking a general overview of the field. The subject matter is wide-ranging and, because the course extends over only one semester, the treatment aims for breadth, understanding, and perspective rather than great depth. The mathematical foundations of the field are deliberately excluded in order to emphasize chemical concepts rather than the traditional engineering or physics treatments. A challenge with this approach is that published material relevant to this subject is widely scattered in specialist books and research articles, and this presents a problem for students who seek to access background reading material. Thus, I have written this book in order to present a qualitative overview of relevant chemistry-related aspects and to provide a springboard to encourage readers to delve deeper into specific topics.
The book begins in Part I with an overview of the elementary chemistry that underlies much of modern materials science. Chapter 1 is a general introduction to the subject, Chapter 2 deals with descriptive ideas of chemical bonding, and Chapter 3 summarizes the background synthesis and reaction chemistry that play a crucial part in many aspects of materials science. Students who are familiar with elementary chemical bonding concepts may wish to move directly from Chapter 1 to Chapter 3. Special characterization techniques that are widely used in materials research are covered in Chapter 4.
Part II focuses on the core of materials chemistry—on different classes of materials, with five chapters dealing with polymers, glasses, oxide and non-oxide ceramics, metals, alloys, and composites. The emphasis in this section is on materials diversity, chemical synthesis, solid-state structure and its relationship to properties, and fabrication methods.
Part III builds on the foregoing chapters and continues with an emphasis on materials that are important in electronics (semiconductors and superconductors), energy-related applications, membranes, optics and photonics, biomedical materials, and an introduction to nanoscience and nanotechnology. Principles of device design and fabrication are increasingly emphasized in this part of the book. A glossary at the end of the book provides a ready reference to the meanings of many of the specialized terms encountered in this field.
Each chapter ends with a brief summary of future challenges in the different fields that could form the basis of class discussions and brainstorming sessions. Also included at the end of each chapter is a list of study questions and suggested reading material for more detailed study. A few of the references are to historical, ground-breaking articles that described major discoveries. Many of the study questions pose practical challenges in new materials design that students are encouraged to address either through written reports or through class discussions. Attempts by students to solve these practical problems have proved to be a popular aspect of the course. This also provides an introduction to the ways in which science and technology become integrated in the wider world.
I am grateful to several colleagues who read sections of the manuscript and made valuable suggestions. In particular, Professor John Badding at Penn State drew my attention to numerous evolving aspects of materials science. Dr. Nicholas Krogman read all the chapters and made many useful suggestions. I am also highly appreciative of the suggestions made by members of my research group and by numerous students who have taken this course and had an opportunity to see preliminary drafts of several chapters. Of course, any errors that remain are mine alone.
I hope that this book proves useful to all who have an interest in the impact of chemistry on modern technology and in the unique ways in which scientists have the knowledge, skills, and vision to bring about dramatic improvements to our way of life.
HARRY R.ALLCOCK
University Park, Pennsylvania
Introduction to Materials Chemistry
Part I
Introduction to Materials Science
1
What is Materials Chemistry?
A. DIFFERENT TYPES OF MATERIALS
Materials science is the science of solids, a field that encompasses most aspects of modern life. This book provides an introductory, qualitative overview of the role of chemistry in several important and expanding areas of materials science, with an emphasis on the ways in which new materials are designed, synthesized, evaluated, and used. It starts from the recognition that there are six or seven different types of solid substances that are the basis of materials science. These are polymers, ceramics, metals, element and interelement semiconductors, superconductors, optical materials, and a range of species in which small molecules are packed into ordered solids (Figure 1.1). These fields were once separate disciplines with little or no exchange of ideas across the boundaries. This is no longer true, and the central area in Figure 1.1 symbolizes research that joins and crosses the different disciplines.
For example, many of the ideas that were developed originally for polymer chemistry are found to explain some of the more puzzling aspects of ceramic science, and vice versa. Concepts that were once thought to be specific to inorganic semiconductors may explain the behavior of some polymers and small molecules in solids. Synthetic diamonds, especially diamond coatings produced by chemical vapor deposition, have characteristics that are reminiscent of both ceramics and semiconductors. Zeolites, which are inorganic materials that contain cavities that accommodate guest molecules and catalyze their reactions, have ceramic network structures but behave like the clathrates formed from small organic molecules in ordered solids. Some ceramics are superconductors, a phenomenon hitherto known only for certain metals and alloys and one inorganic polymer. Thus, a number of the most interesting advanced developments in theory, design, and synthesis are taking place in the central area of hybrid materials shown in Figure 1.1.
Figure 1.1. Different types of materials. Ceramics, metals, semiconductors, and optical materials are traditionally derived from inorganic sources. Polymers and small molecules in solids are normally obtained from organic or organometallic starting materials. The central area represents new types of materials that combine ideas and structures from the traditional areas in order to generate new combinations of properties. It is in this central area that some of the most important future advances can be expected.
This is particularly important because each of the classical materials have advantages and disadvantages. These are summarized in Figure 1.2. For instance, classical ceramics are rigid, chemically inert, and withstand high temperatures, but they are heavy, often brittle, and are difficult to fabricate into complex shapes. Most common metals are strong, tough, and good electrical conductors, but nearly all are heavy and prone to corrosion. There are also serious environmental penalties to be paid for their extraction from minerals and their refining. Inorganic semiconductors play a vital role in communications technology and computing but are difficult to purify and fabricate and are thus expensive. Classical polymers are inexpensive because they are derived from plentiful petroleum. They are easily fabricated because of their low softening temperatures, corrosion resistance, and excellent electrical insulating property. However, most polymers melt at only moderate temperatures, decompose when heated in air, and are flammable. Materials derived from small molecules packed into solids may be semiconductors, but they are brittle and melt at relatively low temperatures. These advantages and disadvantages further illustrate the importance of the central area in Figure 1.1, where cross-disciplinary research aims to produce materials that retain the advantages but minimize the disadvantages of existing materials.
All but two of the main materials areas were once based exclusively on inorganic chemistry. The exceptions were polymer science and small molecules in solids, both of which leaned heavily for their traditions on organic and organometallic chemistry. However, here, too, the boundaries are disappearing, as easily fabricated organic polymeric semiconductors replace traditional silicon semiconductors for some applications, transparent organic glasses replace the heavier silicate-based glasses in lenses, prisms, and some optical waveguides and switches, and ultrastrong polymer–ceramic composites have grown in importance as replacements for metals. Polymers that are part organic and part inorganic have properties that cannot be duplicated by classical polymers, metals, or inorganic ceramics, and these hold considerable promise for the future.
Figure 1.2. Sources and characteristics of traditional materials.
Finally, it is essential for the reader to recognize the fundamental principle that materials science extends across all areas of chemistry, physics, engineering, biology, and medicine, and that breadth of knowledge across the physical and biological sciences is an essential requirement for understanding the materials field.
B. USES OF MATERIALS
The uses of materials are extremely diverse, and a few of them are summarized in Table 1.1 and Figure 1.3. Some fairly obvious applications are as structural materials in automobiles, aircraft, ships, trains, buildings, computer housings, nuclear reactors, and other structures (polymers, metals, and ceramics) where strength, impact resistance, and heat or radiation stability are important.
Other uses are in medical and dental applications that range from instruments, cardiovascular components (Figure 1.3a), drug delivery materials, scaffolds for tissue regeneration, and membranes (metals, ceramics, polymers). Still other applications for materials are in the electrical, computing, and communications sectors [semiconductors, electroluminescent screens, plasma displays, lasers, wiring, insulation. optoelectronic switches, and satellites (Figure 1.3b)]. Finally, a wide range of fibers, films, coatings, membranes, and elastomers are essential components of clothing, automobiles, aircraft, furniture, and manufacturing machinery. Many of these applications cannot be improved unless new materials are discovered that, for example, are fire-resistant, are unaffected by solvents or aggressive chemicals, or remain flexible at very low temperatures.
TABLE 1.1. Example Uses of Advanced Materials in Devices and Machines
Figure 1.3. (a) A prosthetic heart valve with a stainless-steel cage, a silicone rubber ball valve, and a polyester collar for suturing into the damaged organ. (b) The International Space Station showing the silicon semiconductor solar panels and the metal main structure (courtesy of NASA).
Most technology is materials limited. In other words, most advances in technology that are deemed to be desirable or even essential are blocked by the lack of materials that have the right combination of properties. For example, a certain ceramic may be stable to 2000°C and would otherwise be used in gas turbines, but it is too brittle to withstand the shock waves that emanate from the jet engine. A promising polymer has the correct elasticity to be used in replacement heart valves, but its surface characteristics trigger a cascade of reactions that lead to the formation of a blood clot. A superconductor designed for use in levitated trains works well at –150°C but loses its superconducting properties when warmed to room temperature.
C. APPROACHES TO PRODUCING NEW MATERIALS, NEW PROPERTIES, AND USES
There are two different approaches to research and development in materials science. The first is a traditional method used by engineers and many biomedical materials scientists. In this approach, materials are chosen from long-existing solids with a well-known, fixed set of properties that can be changed somewhat by physical manipulation (heat annealing, stretching, incorporation into composites, etc.). Typically this manipulation changes the way in which the molecules or crystallites are organized in the solid state without altering the overall molecular structure. The overwhelming credo is to improve devices or machines by new engineering designs using well-known inexpensive materials rather than more costly new materials. New materials often mean a lot of trouble because engineering and medical protocols have been fixed by long and arduous trial-and-error procedures. Nevertheless, this approach usually must be discarded for critical advanced applications such as aerospace developments or advanced medical devices, where no amount of manipulation of existing materials can generate the crucial combination of required properties.
The second approach is to use chemistry to produce entirely new materials. Chemists are uniquely placed to design and synthesize new materials. Chemists visualize materials down to their structure at the molecular level. They seek to improve materials through molecular chemistry. They ask two related questions: (1) “What is it about the molecular structure of a material that is responsible for its properties?” and (2) “How can I change the molecular structure to improve the materials properties?” This approach is more likely to generate dramatic advances in properties and performance. However, the molecular chemistry approach is successful only if it leads to a marked and often dramatic improvement in properties, because the cost of new products is always high until they can be produced and used on a large scale.
In practice, both the materials manipulation and the molecular chemistry approaches are important, and the challenge is to bring the two together. This connection of chemistry to engineering and medicine is one of the key features of materials science and biomaterials research. It becomes manifest in the sequence of events that occur along the pathway to the discovery and development of new materials. This is illustrated in Figure 1.4. The sequence starts with synthetic and mechanistic discoveries in small-molecule chemistry of the type that all chemists are familiar with. These discoveries can be in organic, inorganic, or organometallic chemistry. In addition, a few of these advances may provide access to new polymers, ceramics, semiconductors, and other materials with entirely new combinations of properties by processes that are described in later chapters. The characterization of these new substances by physical and analytical techniques will provide clues to their possible utility.
Figure 1.4. The sequence of steps from fundamental research to applications.
This second phase accounts for most of the effort that is expended by chemists in new materials research. However, it is not the end of the process, and the third step is critical. In this phase the properties of the new materials are evaluated by physicists, engineers, materials scientists, or medical experts for specific applications. This is the main barrier to utilization because the evaluation procedures are meant to mimic the behavior of the material under operational conditions in an aircraft, a space capsule, a rocket motor or jet engine, or when the material is implanted in a human being. Only if the new material survives these evaluation tests is it allowed to cross the barrier and move into the fourth stage, which is device development and engineering. Concurrent with this final phase, or perhaps preceding it, will be attempts to scale up the synthesis of the material by chemists and chemical engineers so that adequate quantities will be available. Whereas most chemists are pleased to be able to produce 100g of a new compound, applications research normally requires kilogram quantities or more.
D. DEVICES AND MACHINES
One reason why materials science is now such an important part of modern life is because of its influence on the design and operation of devices and machines. Table 1.1 shows some of the modern technologies that are dependent for their continued progress on improvements in materials properties. Energy storage devices such as rechargeable batteries and supercapacitors have reached a plateau in their development because of the sparcity of suitable materials for solid electrolytes. The efficiency of energy generation devices such as fuel cells is seriously compromised by the limitations of the proton-conducting membranes or the ceramic electrolytes on which they depend. These two subjects are discussed in later chapters. A constant need exists for improved semiconductors, especially those that can be fabricated easily and cheaply, with future developments being driven by materials for lightweight flat-panel displays and for imaging and fabrication at the nanometer level. A related area where new materials are needed is in the field of sensors for the detection of microorganisms, toxins, biochemical products, and so on.
The field of optics has for many years depended on the use of inorganic glasses, especially silicate glasses, with various oxides employed to change the refractive index or color. However, irrespective of whether these materials are used in eye-glasses, prisms, camera lenses, or optical waveguides, they are often too heavy and brittle for many applications. For example, the replacement of the electrical circuits in aircraft or automobiles by optical circuits can realize its full potential only if lightweight materials are employed. Thus, the development of lightweight glasses is a major initiative. So, too, is the development of lightweight and easily fabricated materials for photonic switches to replace the semiconductors in electronic circuits.
The medical field has enormous potential for the use of advanced materials. New materials that can break down to harmless products in the body are needed for skin, nerve, and bone regeneration and for the controlled release of drugs. Elastomers that resist bacterial or fungal colonization are needed for artificial heart pumps, blood vessels, and catheters. Membranes for hemodialysis and blood oxygenation still need to be improved. Beyond these well-publicized devices there are many applications that do not appear in the media headlines but on which advanced technology depends.
When confronted by this broad, partial list of needed materials with new property combinations, it is easy to forget that the solutions to these problems lie in the utilization of fundamental chemistry. Without an underlying chemical approach to understanding structure–property relationships, it is unlikely that these applied developments will occur any time soon. Thus, the following chapters are designed to show the reader how chemistry can be utilized for the design and synthesis of both conventional and advanced materials.
E. THE ROLE OF CHEMISTRY IN MATERIALS SCIENCE
Modern chemistry can be divided into the study of two types of substances—small molecules and large molecules. Large-molecule science, together with ultrastructures or “extended lattices” (which are extremely large species in which a visible object consists of one giant molecule), is one of the main foundations of materials science. Small-molecule chemistry (i.e., 2–100 atoms per molecules) is the traditional basis of the chemical sciences, and it is usually the means through which students are introduced to chemistry. Small molecules provide us with most of the insights that underlie our knowledge of ceramics, polymers, and other materials and, as shown in Table 1.2, account for many of the traditional uses for chemicals in modern life. On the other hand, the more complicated molecules and ultrastructures that are used in materials science are the basis for many rapidly advancing technologies such as the other examples shown in Table 1.2. Some solids occupy an intermediate category between small and large molecules. They consist of small molecules assembled in a crystalline lattice in such a way that valuable solid-state properties are generated. These systems also fall into the category of “materials.”
There are two tendencies in modern chemical research. The first is the evolution of chemistry from small molecules to macromolecules, ultrastructures, and materials. The second is the organization of chemical research around properties rather than methodology. Chemistry has a crucial influence on nearly all aspects of materials science. The central role of chemistry stems from the unique ability of chemists to design and synthesize new materials with combinations of properties never seen before. Moreover, together with physicists and engineers, chemists search for a fundamental understanding of why different materials are different and how these differences can be exploited in practical applications.
TABLE 1.2. Comparison of Uses for Small Molecules and Materials
Small Molecules | Materials |
Pharmaceuticals | Structural materials |
Fire retardants | Elastomers |
Dyes | Textile fibers |
Inks | Films and membranes |
Detergents | Surface coatings |
Food additives | Biomedical materials |
Hydrocarbon fuels | Semiconductors |
Insecticides and herbicides | Superconductors |
Chemical warfare agents | Solid ionic conductors |
Optical materials (lenses, optical fibers, NLO materials) | |
Photographic and lithographic materials |
There are three important aspects to modern chemistry. First, a major part of chemistry involves the development of ways to synthesize new compounds, measurement of their properties, and comparisons of their properties with predicted characteristics. It is the synthesis component that distinguishes chemists from nearly all other scientific specialists because there is little point in devising new chemical structures if they cannot be made. The importance of assembling new molecules is illustrated by the relationships shown in Figure 1.5. Thus, pioneering synthesis comes first—without it there are no new molecular structures, new properties, or uses.
The second important aspect of chemical research is to understand the relationship between molecular structure and physical or chemical properties, and to use this information to predict which new molecular structures might have improved properties and uses. Thus, molecular and solid-state structure determination is a key component of materials chemistry research. It follows that structure–property correlations are important as a guide to future work, for example, for molecular and materials design. In a productive research program the sequence of steps involves cycling through the synthesis of new molecules and materials, measurement of molecular and materials properties, and determination of structure, and then using that information as a basis for the design and synthesis of the next generation of materials.
Third, increasingly in more recent years, chemists have become involved in the initial steps to develop practical uses for new materials, often in collaboration with experts in other disciplines. But it is important to recognize that uses are an offshoot of properties, however elegant the method employed for the preparation of the material or its structure determination. Many important developments in materials science occurred before the structure of a substance was known. However, a knowledge of structure is an enormous help in the understanding of both synthesis and properties and therefore for planning future work. It is in this sense that chemistry can be viewed as occupying the central area shown in Figure 1.1, an area that serves as an engine for producing novel materials and processes, from which radiate a flow of new substances that can make possible many diverse applications. The use of the general protocol shown in Figure 1.5 can be put into practice in materials science by answering a number of questions of the type shown in Figure 1.6.
Figure 1.5. Guiding principles of chemistry: (a) pioneering chemical synthesis comes first (without it, there are no new molecules, structures, properties, or uses); (b) structure–property correlations are important as a guide to future work, for example, in molecular or materials design; (c) uses are mainly an offshoot of properties, irrespective of structure or synthesis.
Figure 1.6. Questions that materials chemists must ask.
Many of the following chapters are organized around the subject of materials design, which is the process of integrating synthesis, knowledge of structure–property relationships, and the needs of advancing technologies. It should also be noted that, in both small-molecule chemistry and materials science, attempts to study synthesis, structure, properties, or uses on their own (without integration with the other aspects) lowers the probability that major advances will be made.
F. A BROADER PERSPECTIVE
The scheme shown in Figure 1.7 illustrates how materials chemistry is connected to physics, engineering, biology, and medicine. Chemistry has traditionally been the crucible of new materials for these other areas, and it will continue to be so. It should also be noted that the growing connections between materials science and biology have spawned numerous advances in engineering as well as in medicine.
The further one moves from the fundamental science center shown in Figure 1.7 toward the perimeter, the more it is necessary to take into account the ways in which science and technology interface with the nonscience community. Many potential advances in technology cannot proceed because this outer interface has been neglected or presents an unsurmountable barrier. That barrier may be the high costs needed to produce a new material, it may be the traditions embedded in a particular industry, or it may arise from regulations written into law. Examples abound of new materials that could lead to significant medical, industrial, or aerospace advances, but which have been prevented from doing so by high initial costs or by regulations devised by nonscientists. So science policy, public policy, economics, and manufacturing considerations must always be taken into account as aspects of chemistry move further and further from their intellectual center.
Figure 1.7. Materials chemistry serves as an engine for the design and preparation of new compounds that help the advancement of biology, medicine, physics, and engineering, which are some of the main fields through which chemistry contributes to technology and society.
Figure 1.8. Relationship of materials chemistry to other technical areas and to the wider world.
The early sections of this book deal with the fundamental (and often elementary) chemistry that underlies the main areas of materials science. Subsequent chapters review the synthesis, properties, and fabrication of a wide range of different materials, including ceramics, polymers, metals, small molecules in solids, extended lattices, semiconductors, superconductors, and optical materials. Other chapters describe how the properties of different materials are utilized to bring about improvements in medicine, batteries, fuel cells, and communications technology. Thus, the early chapters are chemistry-oriented. The later ones emphasize devices.
It is hoped that, after reading this book, and contemplating the questions at the end of each chapter, the reader will be able to delve into most of these subjects in greater detail. The references to further reading at the end of each chapter will serve as a starting point for more detailed studies.
G. TERMINOLOGY
One of the hurdles encountered by chemistry students who are entering the field of materials science for the first time is the unfamiliar terminology. This terminology is derived from many technical sources such as ceramic science, polymer science, geochemistry, metallurgy, optics, electronics, and other fields. The Glossary at the end of the book provides explanations of many of these terms. It is recommended that the reader scan this Glossary before proceeding further, and refer to it when an unfamiliar expression appears in the text.
H. EXAMPLE JOURNALS WHERE MATERIALS SCIENCE PUBLICATIONS CAN BE FOUND
ACS Nano [American Chemical Society (ACS)]
Advanced Materials (VCH)
Biomacromolecules (ACS)
Chemistry of Materials (ACS)
Journal of Inorganic and Organometallic Polymers and Materials (Springer)
Journal of Materials Chemistry [Royal Society of Chemistry, London (RSC)]
Journal of Polymer Science—Chemistry and Physics Sections (Wiley-Interscience)
Langmuir (ACS)
Macromolecules (ACS)
Materials Research Society Bulletin (MRS)
Nano Letters (ACS)
Nanomaterials (RSC)
Nature Materials
Science Materials
Soft Matter (RSC)
Solid State Ionics (Elsevier)
Physics Today
I. STUDY QUESTIONS (for class discussions or essays)
1. Wood is a material with outstanding properties, especially strength, impact resistance, and lightweight character. The earliest airplanes were made from wood and canvas, and boats and ships were made from wood until relatively recent times. What is it about the structure of wood that gives it such desirable properties, and why is it no longer used in many common applications?
2. What are the advantages and disadvantages to the production of new materials (a) from petroleum or (b) from minerals? Are there alternatives to these two sources? If so, what are the advantages and disadvantages of these alternative sources?
3. Why is cost such an important factor that determines whether a new material is developed beyond the research stage? What applications can you think of where cost is not a significant factor, and why? Discuss how the cost of a new material might be reduced.
4. It is often said that pioneering research is relatively inexpensive but inherently inefficient, but that the development of uses is efficient but extremely expensive. Why might this be so?
5. Discuss why many engineers and most physicians prefer to use long-established materials rather than new materials that have better combinations of properties. Give examples. What would you do, as an experimental scientist, to help change this situation?
6. What might be the advantages of computers that used lasers instead of electric current, optical waveguides instead of copper or aluminum wires, and very small optical switches in place of transistors?
7. If polymers like polyethylene or polypropylene are lighter than steel, are easily fabricated into intricate shapes, and do not corrode, why do automobile manufacturers continue to use steel for most of their vehicles?
8. The rapid growth of materials science has, if anything, enhanced the need for scientists to have a deeper knowledge of small-molecule fundamental chemistry. Why is this so? Glance at the later chapters in this book before discussing this question.