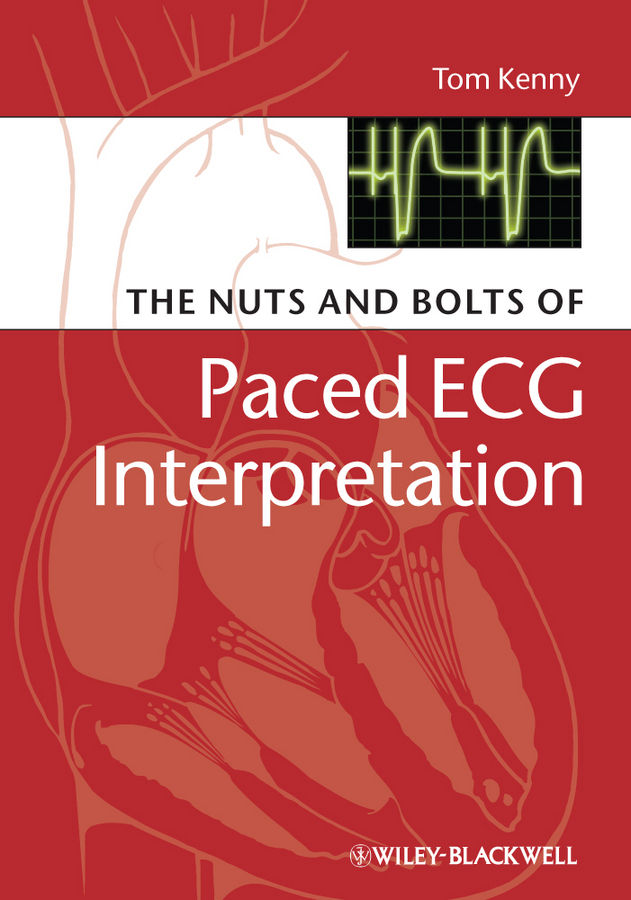
Contents
This edition first published 2009, © 2009 by St. Jude Medical
Blackwell Publishing was acquired by John Wiley & Sons in February 2007. Blackwell’s publishing program has been merged with Wiley’s global Scientific, Technical and Medical business to form Wiley-Blackwell.
Registered office: John Wiley & Sons Ltd, The Atrium, Southern Gate, Chichester, West Sussex, PO19 8SQ, UK
Editorial offices: 9600 Garsington Road, Oxford, OX4 2DQ, UK
The Atrium, Southern Gate, Chichester, West Sussex, PO19 8SQ, UK
111 River Street, Hoboken, NJ 07030-5774, USA
For details of our global editorial offices, for customer services and for information about how to apply for permission to reuse the copyright material in this book please see our website at
The right of the author to be identified as the author of this work has been asserted in accordance with the Copyright, Designs and Patents Act 1988.
All rights reserved. No part of this publication may be reproduced, stored in a retrieval system, or transmitted, in any form or by any means, electronic, mechanical, photocopying, recording or otherwise, except as permitted by the UK Copyright, Designs and Patents Act 1988, without the prior permission of the publisher.
Wiley also publishes its books in a variety of electronic formats. Some content that appears in print may not be available in electronic books.
Designations used by companies to distinguish their products are often claimed as trademarks. All brand names and product names used in this book are trade names, service marks, trademarks or registered trademarks of their respective owners. The publisher is not associated with any product or vendor mentioned in this book. This publication is designed to provide accurate and authoritative information in regard to the subject matter covered. It is sold on the understanding that the publisher is not engaged in rendering professional services. If professional advice or other expert assistance is required, the services of a competent professional should be sought.
The contents of this work are intended to further general scientific research, understanding, and discussion only and are not intended and should not be relied upon as recommending or promoting a specific method, diagnosis, or treatment by physicians for any particular patient. The publisher and the author make no representations or warranties with respect to the accuracy or completeness of the contents of this work and specifically disclaim all warranties, including without limitation any implied warranties of fitness for a particular purpose. In view of ongoing research, equipment modifications, changes in governmental regulations, and the constant flow of information relating to the use of medicines, equipment, and devices, the reader is urged to review and evaluate the information provided in the package insert or instructions for each medicine, equipment, or device for, among other things, any changes in the instructions or indication of usage and for added warnings and precautions. Readers should consult with a specialist where appropriate. The fact that an organization or Website is referred to in this work as a citation and/or a potential source of further information does not mean that the author or the publisher endorses the information the organization or Website may provide or recommendations it may make. Further, readers should be aware that Internet Websites listed in this work may have changed or disappeared between when this work was written and when it is read. No warranty may be created or extended by any promotional statements for this work. Neither the publisher nor the author shall be liable for any damages arising herefrom.
Library of Congress Cataloging-in-Publication Data
Kenny, Tom, 1954-
The nuts and bolts of paced ECG interpretation/Tom Kenny.
p.; cm.
ISBN 978-1-4051-8404-5
1. Electrocardiography. 2. Cardiac pacing. I. Title.
[DNLM: 1. Electrocardiography--methods. 2. Cardiac Pacing, Artificial. WG 140 K365n 2009]
RC683.5.E5K46 2009
616.1’207547--dc22
2008055897
ISBN: 9781405184045
Preface
It seems that every time I start to talk about cardiac pacing and how devices work and how clinicians can better care for the device patients they see, it always comes back to the paced ECG. As much as we like to talk about advanced device features or describe the wonders of downloadable diagnostic reports, I am increasingly convinced that the key to really understanding device behavior is contained in the paced ECGs.
And yet paced ECGs are surprisingly intimidating, even to clinicians who navigate around non-paced ECGs all day long. Pacemakers can behave in unusual and unexpected ways and even when they are behaving themselves appropriately, they can leave a mysterious paced ECG behind for clinicians to deal with.
I wrote this book to supplement the first book in The Nuts and Bolts series, The Nuts and Bolts of Cardiac Pacing. That book, recently revised and updated, contains a lot of good and useful information about how pacemakers work, but it does not take the time to delve thoroughly into the mysteries of the paced ECGs.
Rather than tack this onto the pacing book, I decided to make it a stand-alone volume. Much of the material in this book applies to ICDs with pacing function as well as pacemakers. And because it is its own volume, it can be as thorough as I wanted it to be.
While some clinicians may cringe at seeing a paced ECG, I always like to see them. I like them because most of the time, they tell me that a person is getting improved quality of life and possibly better functional capacity because of a tiny implanted device. I also like them because they can sometimes be a bit of a puzzle. Yet by knowing the basics, keeping it as simple as possible (when in doubt, look at the most obvious solutions), and evaluating everything in a steady, systematic way, most clinicians can learn to interpret tracings accurately.
Early in my career, I was given a great piece of advice with respect to paced ECGs. I was told, “You see only what you look for, you recognize only what you know!” I heard it from one of my first mentors, Dr. Michael Chizner, but the original quotation comes from W. Proctor Harvey, MD, a pioneer of cardiology and professor of medicine at Georgetown University in Washington, D.C. I suspect that many young device specialists struggling to interpret a paced ECG heard that same bit of advice.
It is my privilege, as author of this book, to hand down this sage bit of advice. Don’t let the simplicity of the saying deceive you. It’s a powerful insight that will help you very well as you master the art and science of paced ECGs. “You see only what you look for, you recognize only what you know!”
By the way, paced ECGs (or any tracing) can be surprisingly controversial. That’s one reason they call it ECG interpretation! I have frequently observed formal and informal sessions where respected, knowledgeable, expert colleagues have vigorously disagreed with each other about what a paced ECG might actually mean. The truth is, sometimes we do not know for sure what is going on from an ECG (particularly just one short section of a tracing and viewed apart from the patient). As we developed the workbook section of this book, I saw many ECGs that my colleagues might interpret differently than I do. In the event that you disagree with my interpretations, that’s not entirely unexpected! For my part, I tried to provide evidence for my conclusions so that you can at least follow my thinking. In a few instances, my interpretation is based on “the most likely” interpretation, which, as we all know, may not always be the true one.
I must thank my very wonderful editor at Blackwell, Gina Almond, for her patience and willingness to share my passion for this admittedly unusual subject, and to Kate Newell at Blackwell who does the magic of putting these books together. This is the first time Kate and I have worked together, and it has been a pleasure. As usual, thanks to my creative team who helps translate my ideas into book form. I am indebted to Jo Ann LeQuang (who helps guide the project from my first phone call when I announce, “hey, I’ve got an idea” through the final printing) and Belinda Kinkade (who is one of the few professional artists in the world who can now read paced ECGs). I would also like to thank all of my colleagues at St. Jude Medical who have been overwhelmingly supportive of these books and who are always glad to hear about how useful these volumes have been in your own practice.
As always, I am indebted to my family for their patience as they provided encouragement for me and “another book.” Much of the material for this book has been taken from work done here at St. Jude Medical and I thank my many colleagues for their support and suggestions to make this the best possible book.
Tom Kenny
May, 2009
Austin, Texas
Before We Start …
I wanted to start out this book at ground zero! Whether you are an old hand at ECG interpretation or brand new to the clinical world, ECG interpretation is a challenging practice that can keep you studying – and learning – for an entire career! Paced ECGs are even more of a challenge since the electrical representation of what’s going on in the heart reflects not only intrinsic activity (which can be difficult enough to interpret at times) but also device activity. Device activity changes intrinsic activity and intrinsic activity changes device activity. It can be exciting to see how devices can restore a heart rhythm, but it does not make interpreting paced tracings any easier.
Your job in evaluating a paced ECG is to determine not only what’s going on but to offer a clinical assessment as to the value of what’s going on. Is it helping? Could the device be programmed more appropriately? Is the patient benefiting from the therapy as well as he might? Is the device not “seeing” all that it should see?
The fundamental rule in paced ECG interpretation is this: you see only what you look for, you recognize only what you know.
A secondary rule is that you need to approach any paced ECG systematically. Over the years, I have developed my own system and I am going to inflict that on my readers in this book. But the actual system itself is not as important as the fact that you take a careful, point-by-point approach to looking at any ECG. Don’t skip over things or look at any ECG haphazardly. Too much is at stake for you to approach a paced tracing casually. It can be very tempting to home in on some unusual aspect of a tracing and miss the big picture. That’s why you can’t beat the system when it comes to paced ECGs.
Many of the seemingly simple ECGs you encounter in the clinical setting can turn out to be very challenging, and sometimes an odd-looking ECG is quite clear-cut. Because looks can be deceiving, the systematic approach is invaluable. It will help you uncover troublespots in ECGs that look – at a glance – to be quite normal and it will give you confidence to interpret unusual tracings.
The first portion of this book is going to train you to recognize some of the key landmarks on paced rhythm strips. We’ll go over pacing spikes, paced events, timing cycles, fusion and pseudofusion, and how to determine pacing rates. This entire first section of the book is my attempt to show you the many things that you are going to need to be able to find and distinguish on a paced ECG.
You’ll learn with visual examples. These first chapters are roughly arranged in order of difficulty, but it is my experience as a teacher that those rankings are subjective. If you find a topic particularly challenging, stay with it until you learn the basics.
And with paced ECGs, it always comes back to the basics! It’s all about pacing spikes, sensing, capture, and timing cycles. Just keep those four principles in mind. From there, we’ll build up to rates and special features. It’s a matter of building from the ground up – because you can’t really begin to understand hysteresis behavior until you understand the basics. So please do not rush through the first part of this book, and do not hesitate to revisit it periodically as a refresher.
You’ll also see examples of pacemaker features in action that can complicate the paced ECG. For instance, it’s not unusual in paced patients to see a paced rhythm at a rate above or below the programmed base rate! Oversensing and undersensing are not uncommon on paced rhythm strips. Fusion and pseudofusion have puzzled many clinicians! And if the patient has high-rate intrinsic atrial activity, the pacemaker can demonstrate what we call “upper-rate behavior” or mode switching, all of which show up on the ECG.
Once you know what to look for, you can evaluate most paced ECGs without much trouble, because you are going to recognize what you know.
The second part of the book is my favorite. I’ve found about 100 paced ECGs that I have collected over the years. Most of them are not particularly unusual ECGs. In fact, I’d venture to say that if you worked full-time at a pacing clinic, you’d run into the things illustrated in these strips routinely over the course of a year. I saved these particular strips because they illustrate the very things that clinicians ought to look for (and that means these are the things clinicians must know!). They’re assembled in a workbook format so you can try your hand at evaluating the paced ECG yourself and then read through my systematic analysis on the next pages.
I divided the workbook into sections, rather subjectively classified as easy, moderate, and tough. Then at the very end, I offer a section I called “scramble,” which takes strips of all difficulty levels and offers them in random order. That’s actually the way they will be handed to you if you work in a clinic! You just never know what you will see next.
One last caution. For the purposes of this book, we had an artist re-draw all of our ECGs. We did this mainly for the sake of allowing you to focus on the principles of paced ECG interpretation rather than the vagaries of real-world ECG equipment. In practice, you’ll see strips at different speeds, in different sizes, and you’ll see stuff that can be grainy or blurry. In the real world, you’ll see device artifacts and ECGs that seem to want to fly off the page. Don’t be put off by the fact that the paced ECGs in this book look a little bit too polished or too “perfect.” We did this so you can focus on the basic principles and then apply them to your real-world clinical practice.
Finally, I want to say that I called this book The Nuts and Bolts of Paced ECG Interpretation because “interpretation” is all you can do with an ECG. The clues or evidence that appears on a tracing has to be put together with other factors in order to arrive at a “likely explanation” for what appears on the tracing. Tracings don’t lie, but they aren’t always outspoken with the truth, either. I fully expect that you or other colleagues may occasionally disagree with my interpretation or at least consider a possible ECG interpretation that I dismiss. My interpretation is just that – my interpretation. But it’s based on many years of clinical and industry experience. Over that time, primarily as an educator, I gained a keen appreciation of what seems to trip clinicians up when confronting a paced ECG. Many of these strips were selected with that in mind.
An ECG is just one piece of the puzzle in the care of a pacemaker patient and most ECGs can only tell us about how the patient and device interacted over a few moments of time. Patient symptoms, history, and a routine physical exam are all important in the care of a pacemaker patient. Thus, this book is a bit lopsided in that you will get my interpretations based solely on a strip – something I would not do in the clinic if the patient was available!
And remember: you see only what you look for, you recognize only what you know!
PART I
TIMING CYCLES AND TROUBLESHOOTING REVIEW
SECTION 1
CALCULATING RATES AND INTERVALS
Figure 1.1
Few clinicians can escape their training without having to learn to read a basic ECG. The illustration above is a textbook-type ECG, a perfect example of an ECG that you would probably never see in real-life clinical practice. That’s fine, since you should learn from textbooks and then apply your knowledge to the messier ECGs you’ll come up with in the clinic.
A surface ECG is a graphic representation of electrical energy. The electricity that generates this tracing is obtained from the surface of the skin of the patient. It’s amazing that the heart (about the size of a clenched fist) can generate enough electricity so that it can be picked up on the skin.
The smallest rounded bumps on the ECG are the P-waves, which graphically depict the atrial depolarization. This is followed closely by the largest waveform on the ECG, a pointed wave that goes both below and above the baseline. Called the QRS complex (also sometimes nicknamed an R-wave), this sharp, pointed waveform depicts the depolarization of the ventricles. It’s much larger on the tracing because the ventricles are much larger than the atria and produce more electricity as they squeeze together to pump out blood. After a short pause is another rounded waveform known as the T-wave. The T-wave represents the ventricular repolarization, that is, the time period where the ventricles go back to baseline, in other words, from depolarization to repolarization.
Standard ECG paper has a grid on it that can help you “eyeball” timing. The gridwork is made up of many tiny blocks that are 1 mm square. Each of these tiny boxes is 40 ms duration. Heavier lines are used to make larger squares of five boxes tall and five boxes wide. Each larger square has a duration of 200 ms. Five of these larger squares (200 × 5) equals 1000 ms or 1 sec.
By counting out the grids, you can get a fast approximation of the duration of a particular cardiac cycle or timing cycle. This is going to become increasingly important as we get into paced ECGs. With the heart, timing is everything!
TEST YOUR KNOWLEDGE
1 Looking at this non-paced ECG, would you say that this patient has a regular or an irregular rhythm?
2 Using just this tracing, approximate how long (in ms) the duration is from one P-wave to the next P-wave.
Figure 1.2
This rhythm is regular. Once your eye gets trained to reading ECGs, it is easy to look at a rhythm and appreciate something that is regular or irregular. What makes this rhythm regular, however, is not that the QRS amplitudes are the same or the T-waves have the same morphology (shape). (Those things matter, but for different reasons that we’ll get into later on.) The regularity of this rhythm refers to its even timing. Notice that the complexes all have the same duration. It doesn’t matter whether you measure from P-wave to P-wave, R-wave to R-wave, or T-wave to T-wave, you’ll see the same results. In fact, if you go to the very last complex on the strip, and then added some blank grid paper, you would know enough to draw in where the next P-wave and QRS complex would fall.
In this tracing, we can see that the distance between one P-wave and the next consecutive P-wave is about 1040 ms. That’s based on 4 big squares (4 × 200 = 800) plus 6 little boxes (6 × 40 = 240). You could also measure QRS to QRS or any other complexes. The trick is to measure from the one waveform to the next consecutive waveform of that type.
When clinicians talk about heart rate, we generally use terms like “70 beats per minute” or “82 bpm” or we might say a patient’s heart rate is 120/min. In fact, pacemakers almost always program rate in terms of pulses per minute (ppm) even though this information is not obvious on the ECG. While we humans tend to prefer beats per minute as a way to express rate, pacemakers (and ECG machines) use durations or intervals. That is, this strip does tell us the rate in the form of an interval value (1040 ms). But what rate is that?
Clinicians who work with pacemakers have to be able to convert intervals to rates and vice versa. There are conversion tables that do this and many pacemaker programmers will assist you as well. However, it can also be done with some basic arithmetic or a simple calculator. If one cardiac cycle (i.e., one beat) took 1040 ms, how many beats would occur in a minute? First, we know that 1 minute = 60 seconds or 60,000 ms (60 × 1000). By dividing 1040 (the interval duration) into 60,000 ms (1 minute), you arrive at the rate (57.69 beats a minute, which you would probably state as 58 bpm).
The formula works backward, too. For instance, if you know that a patient’s heart rate is right around 80 bpm, you could expect to see a cardiac cycle interval duration on the ECG of about 750 ms (80 divided by 60,000). That’s three big boxes on the ECG paper and almost four little boxes (3 × 200 = 600 and 4 × 40 = 160).
The nuts and bolts of rates and intervals
SECTION 2
PACING SPIKES
Figure 2.1
This is a paced ECG. You can immediately see it starts to look quite different than a non-paced ECG! The large vertical lines on the strip are pacemaker “spikes,” sometimes called pacing artifacts. This is an electrical signal left on the ECG from the pacemaker output pulse. The output pulse leaves a straight line rather than a more filled out space because a pacemaker output pulse has a very short duration. On average, pacemaker output pulses are about 0.4 ms (that’s less than one thousandth of one second)! That sort of ultra-short but strong energy will just make a line on the tracing rather than a curved or even pointed shape.
These output pulses are quite large. A pacing expert might look at this tracing and tell you the pacemaker was “unipolar.” You’ll also encounter pacemaker spikes that are much shorter (bipolar systems). There can be other reasons for prominent pacing spikes or less visible ones. For instance, the gain control on the ECG monitor may affect the size of the pacing artifact. As a rule of thumb, unipolar pacing involves larger, more visible spikes compared to bipolar pacing. However, new ECG equipment can help adjust the spikes so that unipolar and bipolar spikes appear equivalent.
Unipolar and bipolar are pacing terms that are actually a little bit misleading. They refer to the electrical circuit formed when the pacemaker emits an output pulse. All electricity travels in a circuit, and all circuits have two poles (an anode or positive pole and a cathode or negative pole). A unipolar pacemaker has a lead that forms its electrical circuit using the electrode on the lead itself and the pacemaker can. A bipolar system has a lead with two electrodes that forms a circuit. Thus, the terms “unipolar” and “bipolar” really refer to how many poles are in use on the lead. A unipolar pacemaker has electricity that travels over a wider area than a bipolar pacemaker. As a result, you can expect unipolar pacemakers to show larger pacing spikes than bipolar pacemakers.
The first two QRS complexes on this strip are non-paced. The pacemaker spike appears before the QRS complex in the rest of the complexes. Note that the pacing spike changes the morphology or shape of the QRS complex. This occurs to varying degrees by patient and by the lead observed, but a clinician can often “see” the difference between a paced ventricular depolarization and an intrinsic ventricular depolarization.
There is also an odd-looking event in the middle of the strip. It’s an upward deflection with notches.
TEST YOUR KNOWLEDGE
Figure 2.2
1 Can you see atrial activity on this rhythm strip? Is there atrial pacing going on?
2 How many pacing spikes do you see on this strip? Which chamber of the heart is being paced?
3 Using the grid, approximately how long is the interval between the last pacemaker spike and the one before it?
4 Is that the same as the interval between the first pacemaker spike and the one immediately following it? What rate would this be?
5 What is that odd-looking event in the middle of the strip?
Atrial activity does show up on this ECG but it is not consistent. An imperfect atrial rhythm is nothing unusual, since patients with pacemakers have serious cardiac rhythm problems! The P-wave indicated in the illustration marks an intrinsic atrial depolarization, that is, there is no pacemaker spike to show that a pacemaker output pulse was in any way associated with that atrial event.
There are four pacemaker spikes on this strip. They are large spikes and they show up in association with ventricular events. You can also see that the paced ventricular beats look noticeably different than the intrinsic ventricular events that appear at the beginning of this strip. If you get ECGs with lots of paced and non-paced ventricular activity, it is often possible to use morphology alone to distinguish paced from intrinsic beats.
Looking at the first ventricular spike and the one immediately following it and the last ventricular spike and the one immediately preceding it, the grid work gives us an approximate interval duration of 1000 ms (5 large squares × 200 ms). Converting 1000 ms to a rate is the easiest of all of the interval values: 1000 ms equals 60 beats per minute. The intervals between the two sets of pacing spikes we measured are the same, which shows a nice, consistent pacing rate. (In the clinic, you should check this against how the device was programmed. For this particular patient, expect to see a ventricular pacing rate of 60 ppm.)
In the middle of this strip, there is an odd-looking upward deflection. It looks a lot like a paced ventricular event, but it has a different morphology (shape) and there is a noticeable absence of pacemaker spike. Since this depolarization is not paced, it has to be intrinsic. It appears that about 600 ms (3 large squares × 200 ms) after the previous ventricular output pulse, the ventricles contracted on their own. This is a premature ventricular contraction or PVC. They are not uncommon to see in clinical practice, and they show up on paced ECGs just like non-paced ECGs.
The question with the PVC is this: what does the pacemaker do with it? All pacemakers do only two things: they sense and they pace. We see evidence of ventricular pacing in this strip. Here, with the PVC, we also have good evidence of ventricular sensing. If you try to calculate the interval from the previous pacemaker spike to the PVC, you’ll see that it is much shorter than 1000 ms. That’s because this was a PVC, that is, it occurred much earlier in the cardiac timing cycle. However, once the PVC occurred, the pacemaker should have “sensed” it and used it to reset the pacemaker timing. That is, the distance between the PVC and the next ventricular output pulse should be 1000 ms.
If you measure from the initial upward deflection of the PVC to the next pacemaker spike, you will get five large squares or 1000 ms. This means the pacemaker sensed the PVC, counted it as an intrinsic ventricular event (which it was) and then timed its next pacemaker output to occur 1000 ms later. That is good evidence of appropriate ventricular sensing.
When a pacemaker senses intrinsic cardiac activity that occurs in certain time periods (called “alert periods”), the pacemaker counts the event and uses it as a “landmark” on which to base future timing. In this case, the intrinsic ventricular event (premature ventricular contraction or PVC) caused the pacemaker to withhold a pacemaker output until 1000 ms after the PVC. Had the PVC not occurred, the next pacemaker spike would have occurred much earlier, that is, 1000 ms after the previous spike. The ability of the pacemaker to withhold pacing when events are sensed is known as “inhibition.” Here, a PVC occurred which inhibited the ventricular output until the appropriate interval timing could be restored.
The nuts and bolts of pacing spikes
SECTION 3
THE BASICS OF CAPTURE AND SENSING
Figure 3.1
This paced ECG shows atrial and ventricular activity, but notice where the pacing spikes fall. This is an ECG from a device pacing the atrium. In the very first complex, there is an atrial spike, an atrial event, and an intrinsic ventricular event. It happens again in the second complex. But now look at the third complex.
In this particular complex, the pacing spike falls right in the middle of the ventricular complex. The QRS complex is not paced; it has the same morphology as the intrinsic QRS complexes that preceded it. What’s going on? The spike occurs on the atrial channel and although it occurs at the same time as the ventricular event, it is unrelated to the intrinsic ventricular event.
The next pacing spike occurs after an intrinsic ventricular event. Then there is another “displaced” spike. In terms of what is going on in the heart, the spikes seem to just occur at random. But take a closer look! It isn’t random at all. Measure the distance between the spikes. You can eyeball it or get out the calipers. You’ll be able to count out 840 ms between spikes (4 large squares and 1 small box or 4 × 200 = 800 + 40 = 840 ms) which converts to about 71 beats a minute.
This pacing behavior is not random at all; the atrial spikes appear at precise intervals. However, they are not synchronized or working with the intrinsic activity.
This strip is an example of what we would call an asynchronous pacing mode. It’s AOO pacing. The device paces the atrium but it does not sense; in fact, is has no regard for intrinsic activity.
In clinical practice, you won’t see asynchronous pacing very much, but it can be used for testing, backup pacing, and may be used in certain unusual situations. You should be aware of what asynchronous pacing looks like, though! In this strip, it is appropriate since the device is programmed to AOO pacing, and this is clearly AOO pacing. In most instances, however, clinicians would want to avoid this kind of pacing.
By the way, “appropriate” is a favorite word for pacing experts because it refers to what the device “ought to do.” Since this pacemaker was programmed to AOO pacing, the above strip is absolutely appropriate. However, if the pacemaker was programmed to AAI pacing and this strip appeared, there would be a problem. That’s why, as a clinician, it is always useful (even if not always possible) to know the pacing mode and other programmed settings.
By the way, appropriate means the expected behavior of the device as programmed. Sometimes a pacemaker performs appropriately, but it is not necessarily the optimal pacing therapy for the patient. This strip might be a good example of pacing that is appropriate, but not optimal!
TEST YOUR KNOWLEDGE
1 Would you say that there is atrial capture going on in this strip? If so, where?
2 Would you say that there is atrial sensing going on in this strip? If so, where?
3 How would you explain the last complex in this strip?
4 Is there any ventricular pacing going on in this strip?
5 If somebody were to ask you to “prove” this strip showed asynchronous pacing, how would you prove it?
Atrial capture occurs when there is an atrial pacing spike that causes an immediate atrial depolarization, which would show up on the strip as an atrial event (P-wave). The first, second, and next-to-last complex all show an atrial pacing spike and an immediate atrial depolarization. But was it capture? It is what a pacing specialist might call “apparent capture.” That is, it looks like capture but it is hard to know for certain.
The reason that it is “apparent capture” rather than “definitely capture” is that the strip shows other intrinsic atrial activity for this patient. The atria are beating on their own, although not regularly and not every complex. So it is possible that a pacing spike just happened to occur precisely at the moment the atria depolarized. From this tracing by itself, it is just not possible to be sure there was true capture.
Atrial sensing means that the pacemaker would “see” intrinsic atrial activity and then adjust pacing behavior. That is not happening. For instance, the fourth pacing spike falls relatively quickly after an intrinsic atrial event. Had the pacemaker “seen” that intrinsic atrial event, it would have inhibited the atrial output.
You can also get a clue that there is no sensing going on by the fact that although the patient’s rhythm is a bit erratic, the pacing spikes appear with military precision every 840 ms. When a pacemaker is sensing, you will only see such exact pacing spikes when the patient has a very stable rhythm. (By the way, since this is AOO or asynchronous atrial pacing, you also know from the mode that there is no sensing. This is covered in the next “In Depth” section.)
The last complex in this strip looks a bit strange. The event is a PVC. However, the device delivers an atrial output pulse at the same time. On the ECG, they appear together, but the PVC is an intrinsic ventricular event while the spike occurs on the atrial channel.
If you were to just have one or two complexes of this tracing, it might be hard to say if the pacing spikes were atrial or ventricular. That’s because the ECG captures all electrical activity in both chambers and reports it in one tracing. You can’t readily see whether the spike is coming from an electrode in the atrium or the ventricle! But if you look at the larger picture of several complexes, you can see that there is apparent atrial capture in three of the complexes and no examples of apparent or even possible ventricular capture in the rest of the strip.
Figure 3.2
But the real clincher that shows this is an AOO and not a VOO system is the third complex. If a ventricular output pulse actually occurred in the middle of a ventricular intrinsic beat, it would very likely distort the QRS morphology. The distortion may not be dramatic, but the QRS would show some electrical impact. Since that isn’t going on here, the evidence strongly suggests that the spike is atrial.
If you knew the patient had an atrial pacemaker but you did not know the mode and suspected asynchronous pacing, you could demonstrate AOO mode by measuring the distance between pacing spikes. Since asynchronous pacemakers do not sense, there is nothing to change the timing cycles. Pacing spikes appear at regular intervals with no deviation, even if the patient’s rhythm is unstable. Further evidence is the fact that intrinsic atrial events do not seem to impact the timing of atrial pacing. If the device could sense atrial activity (and was not sensing inappropriately), the fourth pacing spike would have been greatly delayed. This is almost “textbook” atrial asynchronous pacing!
The nuts and bolts of capture and sensing
SECTION 4
IN DEPTH: MODES
Whenever you see paced ECGs, it is always a good idea to get as much information as possible about how the pacemaker is programmed. One of the most basic and useful bits of information to know is the pacing mode. Mode refers to how the pacemaker is set up to pace and sense cardiac activity.
Single-chamber modes have pacing function only in one chamber, typically the ventricle. Atrial single-chamber pacemakers do exist, but are not as common to see in clinical practice as ventricular single-chamber pacemakers. Dual-chamber pacemakers pace in both chambers. In many practices today, dual-chamber pacemakers are the most typical device you will encounter. Dual-chamber pacing is a bit more complex than single-chamber pacing and will be covered in more detail in later chapters.
The pacemaker code in common use provides three or four letters that are shorthand for how the device functions. The first letter shows where the device paces, the second letter shows where the device senses, and the third letter shows what it does if it senses an event. If the device offers rate response (covered in a later chapter), the fourth letter will be an R. If there is no fourth letter, you should assume the device is not rate responsive.
A VVI pacemaker paces in the ventricle (V), senses in the ventricle (V) and when it senses in the ventricle, its response is to inhibit (I) or withhold an output pulse.
An AAI device is the same thing, but in the atrium.
VVT pacing refers to a device that paces in the ventricle (V), senses in the ventricle (V), but when it senses something in the ventricle, its response will be to track (T) or pace in response. Tracking means that an output pulse is required of the device. AAT is the atrial counterpart to VVT mode.
For dual-chamber devices, the most common annotation is DDD or DDDR. A DDDR device paces in both chambers (D or dual), sensed in both chambers (D) and when it senses something may either inhibit or track (D for dual response) and it has rate response (R).
The “either inhibit or track” response conveyed by the third D in DDD is a bit tricky. If the pacemaker senses an intrinsic atrial event, it will inhibit an atrial output pulse; likewise, if it senses an intrinsic ventricular event, it will inhibit a ventricular output pulse. However, if the DDDR pacemaker senses an atrial event, it will force a ventricular output if an intrinsic ventricular event does not occur at the proper time (“tracking”). The purpose of atrial tracking is to allow the pacemaker to provide 1:1 AV synchrony (one atrial event matched to one ventricular event), even if the patient’s intrinsic atrial rate goes above the programmed pacing rate. Thus, a DDD pacemaker that senses an atrial event will withhold the next atrial output pulse (inhibition) but may pace the ventricle in response (tracking).
All of these types of pacing could be called “synchronous” pacing. An older term for synchronous pacing was “demand pacing” (the pacemaker paced when the patient’s heart required or demanded it). Synchronous pacing means that the pacemaker senses cardiac activity and attempts to fill in the missing beats only if necessary. Synchronous pacing (VVI, VVIR, AAI, AAIR, VVT, AAT, DDD, DDDR, DDI, DDIR, etc.) might be thought of as pacing that tries to sync up or time itself to work with the patient’s intrinsic activity. Synchronous pacing always involves both pacing and sensing.
Asynchronous pacing is pacing without sensing. When you see asynchronous pacing, there will be regular pacing spikes but they may fall in strange places with regard to the patient’s own rhythm. There is no attempt to sync pacing activity to the patient’s intrinsic cardiac activity. As a general rule, asynchronous pacing is not beneficial for most patients and is rarely, if ever, used. Asynchronous modes are available for testing purposes and special circumstances. They are AOO, VOO, and DOO plus their rate-responsive counterparts, AOOR, VOOR, DOOR.
Mode | What it does | What to expect on the ECG |
VOO | Asynchronous ventricular pacing | Regular ventricular pacing spikes without any regard to intrinsic activity |
VVI | Paces and senses the ventricle | Ventricular capture (with widened QRS morphologies), ventricular inhibition when a ventricular depolarization occurs on its own |
VVT | Paces the ventricle at regular intervals or when an intrinsic ventricular event occurs | Ventricular capture (with widened QRS morphologies) at regular intervals – this happens when there is no intrinsic ventricular depolarization. If intrinsic ventricular depolarizations occur, you’ll see a somewhat different QRS morphology with a spike in it. These may occur at more irregular intervals (matching the patient’s intrinsic ventricular rate) |
AOO | Asynchronous atrial pacing | Regular atrial pacing spikes without any regard to intrinsic activity; it may not be immediately clear if the spikes are atrial or ventricular unless you can see several complexes |
AAI | Paces and senses the atrium | Apparent atrial capture and atrial inhibition when an intrinsic atrial event occurs |
AAT | Paces the atrium at regular intervals or when an intrinsic atrial event occurs | Apparent atrial capture at regular intervals – this happens when there is no intrinsic atrial depolarization. If intrinsic atrial activity occurs, you’ll see atrial events with a spike. These may occur at more irregular intervals (matching the patient’s intrinsic atrial rate) |
DOO | Paces and senses both atrium and ventricle but does not sense | Two spikes per complex, with atrial and ventricular spikes occurring at exact intervals with no relation to the patient’s own rhythm |
DDI | Paces and senses both atrium and ventricle but will inhibit pacing when an intrinsic depolarization occurs | This is a fairly complex ECG which should show atrial capture or sensed atrial activity and ventricular capture or sensed ventricular beats. If the patient has intrinsic atrial activity above the programmed base rate, the ventricles will not be paced to keep up with the native atrial rate. |
DDD | Paces and senses both atrium and ventricle but will inhibit pacing when an intrinsic depolarization occurs in one chamber; if it senses an atrial event, it will require the ventricle to depolarize, either by pacing it or sensing an intrinsic event within a certain timing cycle | This paced ECG is both complex and quite common in clinical practice. It will usually show pacing activity going on in both atrium and ventricle. You will often see paced atrial events and paced ventricular events. If sensed events appear, you should see how they were “taken into account” by the pacemaker; in other words, the device inhibits a ventricular spike if there was a native ventricular event. One unique feature of a DDD-paced ECG is that in the presence of rapid intrinsic atrial activity, you may see rapid ventricular pacing, even at rates above the base rate! This is atrial tracking – and we’ll cover it more in depth in later sections |
How do you find out the mode? If you have access to a pacemaker programmer and can download programmed settings, the pacemaker’s mode will be prominently displayed in most diagnostic and general reports. If the patient carries a pacemaker ID card or other information from his or her doctor, the pacemaker mode may be stated. Pacemaker ID cards state the highest mode to which the pacemaker can be programmed, and this is usually (but not necessarily) how the device is programmed. (A DDDR pacemaker can be programmed to pace as a DDD or DDI or even a VVIR or VVI device! However, a VVI device cannot be programmed “up” to DDD since it only has one lead.)
If you cannot figure out the mode but know the manufacturer, you can call the Technical Services hotline of the manufacturer and ask about the mode for that particular model of pacemaker. Otherwise, the clinician has to “deduce” the mode by looking at clues on the ECG. Believe it or not, with some practice, most clinicians get fairly adept at recognizing classic pacemaker behavior. By far the most common pacing modes are VVI and VVIR for single-chamber devices and DDD and DDDR for dual-chamber devices.
Modes are programmable, which means that physicians are free to program what they deem the most appropriate mode for a patient. While this is not common practice, it is not unusual for a mode to be changed in response to a clinical situation. For instance, a patient with a DDD device may be reprogrammed to VVI pacing if he has atrial fibrillation. Thus, even if you think you know the mode of the device, you should still look carefully at the paced ECG.
The nuts and bolts of modes in depth